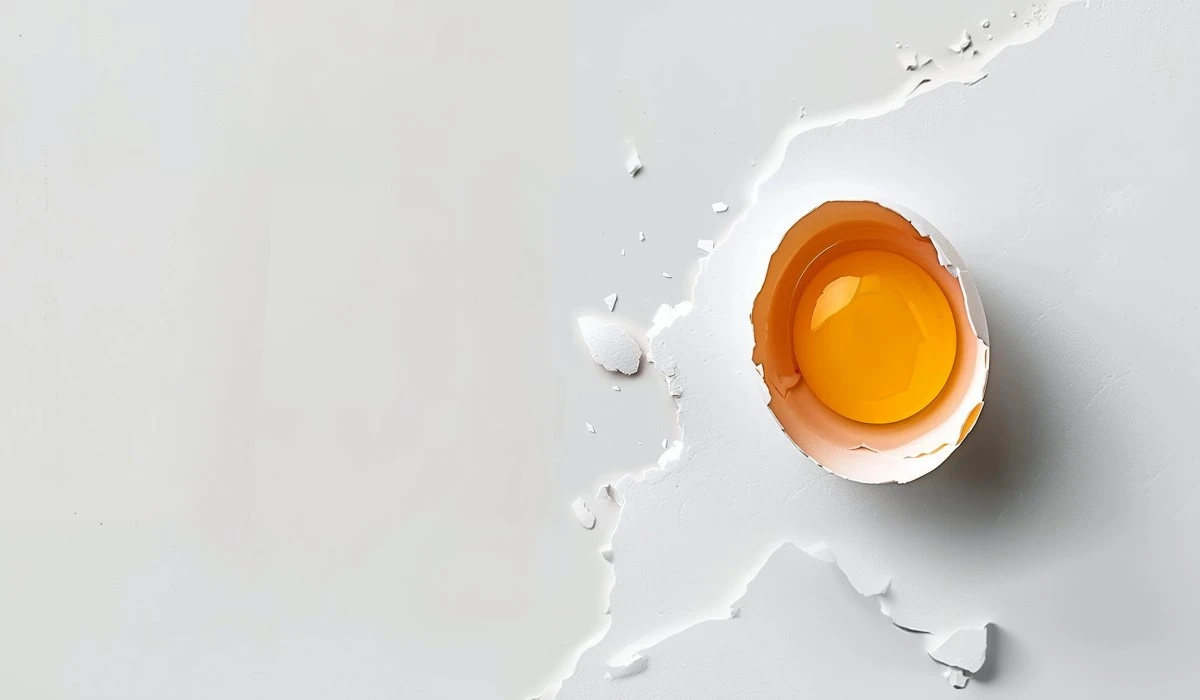
MIT Cracks Egg Drop Science Myth
Cracking the Code: The Surprising Truth About Falling Eggs
A familiar educational exercise for countless science students involves dropping eggs. This activity sees participants attempt to protect a fragile hen's egg from shattering after a significant descent. Typically, instructors use this project to demonstrate fundamental physics concepts. Generations of pupils absorbed a specific piece of 'common sense' wisdom during this particular exercise. The prevailing advice indicated an egg displays greater strength when impacting the ground vertically, on one of its pointed tips. Recent meticulous research, however, casts serious doubt upon this long-held assumption. Engineers affiliated with the Massachusetts Institute of Technology (MIT) conducted rigorous tests. Their conclusions indicate the opposite might actually be accurate. This surprising discovery alters our understanding of eggshell mechanics and also offers valuable lessons concerning scientific inquiry itself. It reminds us to scrutinise even seemingly obvious notions.
A Classroom Staple Under Scrutiny
The activity involving falling eggs serves as a practical introduction to physics principles for many learners. Students receive a raw egg alongside access to various materials like cotton wool, sticky tape, straws, or cardboard. Their objective requires constructing a protective casing or structure. This device must adequately shield the egg to prevent breakage upon impact after release from a considerable elevation, frequently a school roof or ladder. The assignment highlights concepts like kinetic energy, impact force, momentum transfer, and structural design. Participants swiftly realise the substantial difficulty involved in engineering an effective protective solution. Resulting messy outcomes for less successful designs often remain vivid memories. Traditionally, teachers explained that orienting the egg vertically within the contraption offered the premier survival chance, capitalising on the supposed strength originating from the egg's pointed ends. This advice felt intuitively correct, seeming to follow from the structural strength of an arch shape when stationary.
The Enduring Myth of Vertical Strength
The belief that an egg better withstands force along its vertical axis has persisted for decades. Science communicators, educational materials, and even online resources frequently echo this assertion. The reasoning appears logical when one considers static forces, like gently squeezing an egg. The curved shape of the tips resembles an arch, a famously robust structural form capable of effectively distributing pressure. Many people assumed this static resilience directly translated to dynamic impact scenarios, such as following a drop. This 'common sense' conclusion suggested that landing tip-first would permit the eggshell to better resist the sudden shock. Consequently, students taking part in exercises with falling eggs received routine advice to position their eggs upright within protective designs. Commercial egg packaging also often utilises this vertical orientation, potentially reinforcing the perception of end-on strength. This widespread conviction formed the strategic bedrock for innumerable attempts globally.
Image Credit - Freepik
Questioning Convention at MIT
Established wisdom surrounding egg drop physics eventually encountered scepticism at a leading engineering institution. Tal Cohen, an associate professor working within the Department of Civil and Environmental Engineering at the Massachusetts Institute of Technology, developed reservations. Professor Cohen regularly supervises an egg-dropping competition as an orientation component for first-year engineering students at MIT. While annually explaining the conventional advice about vertical orientation, she began wondering if this truly depicted the dynamic reality of an object in descent. The assertion seemed principally derived from observations concerning how eggs behave under steady, stationary pressure, not the sudden, complex forces intrinsic to an impact. This vital distinction prompted Cohen alongside her research group to investigate further, advancing beyond established classroom narratives to pursue empirical evidence. Their scientific curiosity motivated them to transfer the seemingly simple classroom query into the controlled laboratory setting.
Designing the Crucial Experiment
To rigorously assess the common assumption, Professor Cohen's group initiated a carefully planned sequence of experiments. They first acquired a significant quantity of hen's eggs – sources mention a figure exceeding 200 specimens – from a local Costco wholesale outlet. The researchers subsequently subjected some eggs to static compression trials employing specialised equipment. Using this apparatus enabled precise measurement concerning the force magnitude needed for shell failure when pressure increased slowly, comparing vertical (tip-on) and horizontal (side-on) orientations.
Following these static evaluations, the team progressed to dynamic drop experiments. They assembled a specialised rig involving solenoids and 3D-printed holders, ensuring consistent egg release from specific heights with controlled impact orientations. Critically, they selected very low drop heights – starting at just eight, nine, and ten millimetres – onto a rigid surface. This minimal falling distance permitted observation across a spectrum of outcomes, including non-breakage, which greater drop heights would likely obscure by shattering all eggs independent of positioning.
Measuring the Force to Fracture
The investigation's initial phase comprised static compression assessments. Researchers positioned individual eggs within equipment applying a gradually mounting force. They meticulously logged the precise force level necessary to induce the shell's first crack. These tests compared eggs situated upright (vertically) against eggs resting horizontally. Findings from this static analysis proved somewhat unexpected. Measurements indicated that a broadly similar force magnitude – approximately 45 Newtons – caused eggshell fracture, irrespective of whether force application occurred at the tips or the sides. This result directly challenged the intuitive notion that the tips possessed inherent superiority under slow, constant pressure. Although the ultimate breaking force demonstrated similarity, researchers did note a subtle distinction in pre-failure deformation behaviour, suggesting variations in shell compliance.
The Dynamic Drop Difference Revealed
Truly counter-intuitive outcomes materialised during the dynamic drop evaluations. The MIT group carefully dropped 180 eggs from controlled, minimal heights (8mm, 9mm, 10mm), guaranteeing equal numbers impacted vertically and horizontally. Results sharply contrasted with established 'wisdom'. Eggs released vertically, striking either pointed tip, fractured much more often, even from these minuscule heights. As an example, from merely 8mm, over half the vertically dropped specimens cracked following impact. In dramatic opposition, fewer than ten percent of eggs dropped horizontally, making contact while positioned sideways, fractured from the identical 8mm height. This statistically meaningful disparity demonstrated unequivocally that, concerning dynamic impact situations, the horizontal alignment provided substantially superior fracture protection. The egg's side proved considerably more resilient against the abrupt landing shock.
Image Credit - Freepik
Flexibility Versus Stiffness: The Key Insight
Researchers investigated more deeply to comprehend why horizontal drops proved advantageous. The explanation resides in the differing eggshell responses to impact contingent on orientation. When an egg strikes the ground on its side, the shell displays greater compliance or flexibility. It can deform slightly inwards upon collision, compressing more than the tips manage before fracturing. This minor bending functions akin to a shock absorber, effectively dispersing part of the impact energy over a fractionally extended duration and across a broader area. Conversely, the egg's pointed tips possess greater structural rigidity. While stiffness might appear beneficial, during a dynamic impact, this inflexibility means the tips cannot yield sufficiently to cushion the shock. Impact force concentrates intensely at the contact point, leading more readily to fracture. This observation highlights a crucial distinction separating stiffness (deformation resistance) and toughness (pre-break energy absorption capacity).
Learning from Our Knees: A Human Analogy
Joseph E. Bonavia, an MIT graduate student participating in the research, presented a useful analogy comparing the egg's actions with human anatomy. He observed that if an individual tumbles from elevation, instinctively locking their knees straight poses danger. Rigidly locked legs directly transmit the impact shock upwards through bones, elevating fracture risk. Conversely, bending knees upon landing permits leg muscles and joints to absorb impact energy more progressively. This flexing motion effectively pads the blow, safeguarding the skeletal structure. Bonavia elucidated that the horizontally aligned egg operates analogously to bent knees. Its capacity for slight compression on its side enables more effective absorption and distribution of impact force, boosting its toughness and diminishing catastrophic failure probability, just as flexed knees shield a falling individual. This comparison renders the complex physics more readily understandable.
Kitchen Habits and Misconceptions
Another study contributor, MIT graduate student Brendan M. Unikewicz, advanced an intriguing hypothesis regarding why the vertical strength myth gained such traction. He posited that our daily routines cracking eggs for culinary use might contribute. Most individuals initiate cracking by tapping the egg's midpoint – its side or 'equator' – against a firm surface like a bowl rim or worktop. This action typically generates long, clean fissures, perfect for neatly splitting the shell into halves to release contents for scrambling or frying. Witnessing the side yielding so easily in domestic settings could inadvertently bolster the conviction that this section represents the weakest point. However, the sharp, localised force exerted during cooking differs markedly from forces encountered during a fall. Laboratory experiments unequivocally illustrated that impacting the tips prompted shell collapse inwards rather than clean splitting – an unsuitable cooking result but indicative of a distinct failure mode under collision conditions.
The Underlying Physics of Impact
The MIT investigation elegantly showcases fundamental physics concepts concerning impact phenomena. When an object descends, it carries kinetic energy owing to its motion. Upon striking a surface, this energy requires rapid dissipation. Force experienced during collision depends critically upon the deceleration rate as the object halts. This connection involves the impulse concept – the momentum change. By lengthening the timeframe over which deceleration transpires, peak impact force diminishes significantly, even while total absorbed energy stays constant. The horizontally falling egg accomplishes this through flexing. Its slight compression prolongs impact duration compared to the abrupt stop encountered by the stiffer, vertically impacting egg. This temporal force distribution represents the key to its enhanced resilience against fracturing during a descent.
Inside the Eggshell: A Natural Bioceramic
Comprehending eggshell material characteristics offers additional perspective. An eggshell consists primarily of calcium carbonate, arranged in a particular crystalline configuration termed calcite. This composition classifies it as a natural bioceramic type. Ceramics generally exhibit hardness and stiffness (possessing a high Young's modulus) but concurrently display brittleness, meaning they typically fracture abruptly rather than deforming substantially (low fracture toughness). Research substantiates that eggshell aligns with this profile, demonstrating notable stiffness relative to its thickness alongside very low fracture toughness compared with other natural calcium carbonate structures like seashells. This distinctive blend suits its biological function: furnishing a rigid, protective enclosure for the developing chick while remaining sufficiently brittle for hatching emergence. Nevertheless, this inherent brittleness renders it vulnerable to fracture originating from microscopic flaws, particularly under impact.
Image Credit - Freepik
Engineering Safer Landings: Crumple Zones
The principle involving impact energy absorption via controlled deformation, exemplified by the sideways-descending egg, finds direct analogues in contemporary engineering, notably within vehicle safety design. Automobiles integrate 'crumple zones' – designated front and rear regions engineered for predictable deformation and collapse during a collision. These zones behave sacrificially, absorbing a considerable crash energy fraction through crushing, much like the flexing eggshell side. This mechanism extends the car's deceleration period, drastically curtailing peak forces reaching the rigid passenger cell and occupants. Engineers employ sophisticated materials and structural configurations, encompassing multi-chamber extruded aluminium profiles or energy-absorbing foams, optimising zone collapse behaviour and impact force management, ultimately improving passenger survival prospects.
Protecting Precious Cargo: Packaging Insights
The task of safeguarding fragile articles during shipment mirrors the falling egg experiment on a commercial footing. Protective packaging strategies rely heavily upon identical impact absorption and force distribution tenets. Materials such as bubble wrap, foam inserts (polystyrene, polyurethane), corrugated cardboard frameworks, and even moulded pulp (akin to egg cartons) function to cushion delicate contents. These materials operate by forming a buffer that yields upon impact, lengthening the packaged item's stopping distance and duration. This action lessens deceleration forces acting upon the product, averting damage. Just as the horizontally positioned egg's compliance conferred superior protection, packaging engineers choose materials and configurations offering appropriate cushioning levels – sufficient flexibility for shock absorption without excessive softness permitting contact with the outer container. Managing kinetic energy transfer safely remains the constant objective.
Helmets and Hard Hats: Personal Protection
Impact absorption science extends pivotally to personal protective gear like helmets utilised in sports, construction, and motorcycling. A helmet's core purpose involves shielding the head from injury during impact. It accomplishes this via multiple cooperative mechanisms. The rigid outer shell distributes initial impact force across a broader surface. Beneath this shell resides a compressible foam liner layer, frequently expanded polystyrene (EPS). This liner functions as the crucial energy absorber. Upon impact, the foam crushes and deforms, comparable to an automobile's crumple zone or the yielding eggshell. This managed deformation absorbs substantial impact energy, decelerating the head more gradually and reducing forces conveyed to the brain. Helmet efficacy fundamentally depends on this liner's capacity for impact energy management through controlled failure, underscoring toughness's importance over simple rigidity.
Image Credit - Freepik
Lessons for the Laboratory and Beyond
Professor Cohen stressed these conclusions possess wider relevance beyond merely comprehending eggs. The research acts as a potent reminder concerning empirical testing's importance and potential hazards linked to relying solely upon intuition or 'common sense' within science and engineering. What appears intuitively valid under static circumstances might not persist in dynamic scenarios. The investigation underscores the imperative for engineering professionals and scientists to maintain curiosity and readiness to challenge long-established presumptions, including those widely taught. Demonstrating courage to query conventional wisdom via rigorous experimentation constitutes a fundamental scientific progress element. This cycle involving testing, observing unexpected outcomes, and revising comprehension remains central to knowledge advancement across all technical domains.
Updating the Science Lesson
The MIT group's finding invites reconsideration regarding how the classic falling egg activity features in educational contexts. While the exercise persists as a valuable instrument for imparting physics and engineering design concepts, educators now possess more precise data concerning optimal egg orientation for impact survival. Focus might perhaps shift subtly. Rather than merely transmitting the (now discredited) vertical strength myth, instructors can leverage this research as a case study. It illuminates the disparity between static stiffness and dynamic toughness. It showcases experimental verification's power over untested beliefs. The challenge evolves into an undertaking not solely focused on building protective apparatus, but also on employing the scientific method for testing variables like alignment and grasping impact physics nuances.
Nature's Design and Dynamic Forces
Although the eggshell demonstrated superior resilience against drops when impacting sideways, its natural configuration likely gives precedence to alternative factors. The overall ovoid form delivers structural soundness against relatively consistent, static loads encountered within a nest – for instance, the parent bird's weight during incubation. The arch-like tips suit this static pressure distribution well. Evolution probably did not optimise eggshells explicitly for surviving significant falls, an infrequent event in natural nesting compared to constant static load bearing needs and eventual chick emergence. The shell's low fracture toughness, paired with high stiffness, mirrors this equilibrium – sufficiently strong for protection, adequately brittle for necessary breakage. The MIT research concentrates specifically on dynamic impact physics, uncovering properties less pertinent to the egg's standard ecological setting but critical for engineering uses.
Image Credit - Freepik
The Fate of the Experimental Subjects
A practical query emerges regarding the numerous eggs employed during experimentation. Institutional regulations at MIT, common across research establishments, forbid human consumption of materials once introduced into laboratory settings due to potential contamination or procedural mandates. Consequently, researchers themselves could not partake in omelettes prepared from their study subjects. However, the account suggests Professor Cohen's canine companion, unbound by identical regulations governing human researchers, derived benefit. The dog reputedly consumed several substantial meals comprising post-experiment eggs, guaranteeing the biological matter avoided complete wastage after fulfilling its scientific role. This small detail imparts a touch of relatable realism to the demanding scientific endeavour.
Beyond the Breakfast Table: Wider Implications
Principles examined within the falling egg investigation resonate throughout numerous scientific and engineering fields. Understanding structural responses to dynamic impacts proves critical across domains from aerospace engineering (designing landing spacecraft or resisting micrometeoroid strikes) to civil engineering (ensuring structures withstand earthquakes or collisions) and materials science (creating novel materials featuring enhanced toughness and impact resistance). Fundamental energy absorption, force distribution, stiffness versus toughness concepts, and material failure mechanisms possess universal applicability. The humble egg, via this meticulous inquiry, furnishes a surprisingly potent model for exploring these intricate phenomena, demonstrating that valuable discoveries frequently arise from examining commonplace objects with renewed scientific scrutiny.
A Final Crack at the Problem: Challenging Assumptions
In conclusion, research undertaken at MIT effectively refutes a durable piece of conventional wisdom pertinent to the popular egg dropping activity. Experiments conclusively show eggs possess a reduced fracture likelihood when dropped horizontally striking their sides, rather than vertically impacting their tips. This counter-intuitive outcome originates from the enhanced flexibility and energy-absorbing capability inherent in the eggshell's side relative to its stiffer tips under dynamic impact conditions. Beyond rectifying a prevalent classroom misconception, the study champions core scientific inquiry values: questioning assumptions, grounding conclusions in empirical data, and acknowledging the crucial divergence between static properties and dynamic behaviour. It serves as a compelling illustration that even elementary phenomena can reveal surprising truths following rigorous investigation, yielding implications extending into complex engineering design and material science realms.
Recently Added
Categories
- Arts And Humanities
- Blog
- Business And Management
- Criminology
- Education
- Environment And Conservation
- Farming And Animal Care
- Geopolitics
- Lifestyle And Beauty
- Medicine And Science
- Mental Health
- Nutrition And Diet
- Religion And Spirituality
- Social Care And Health
- Sport And Fitness
- Technology
- Uncategorized
- Videos