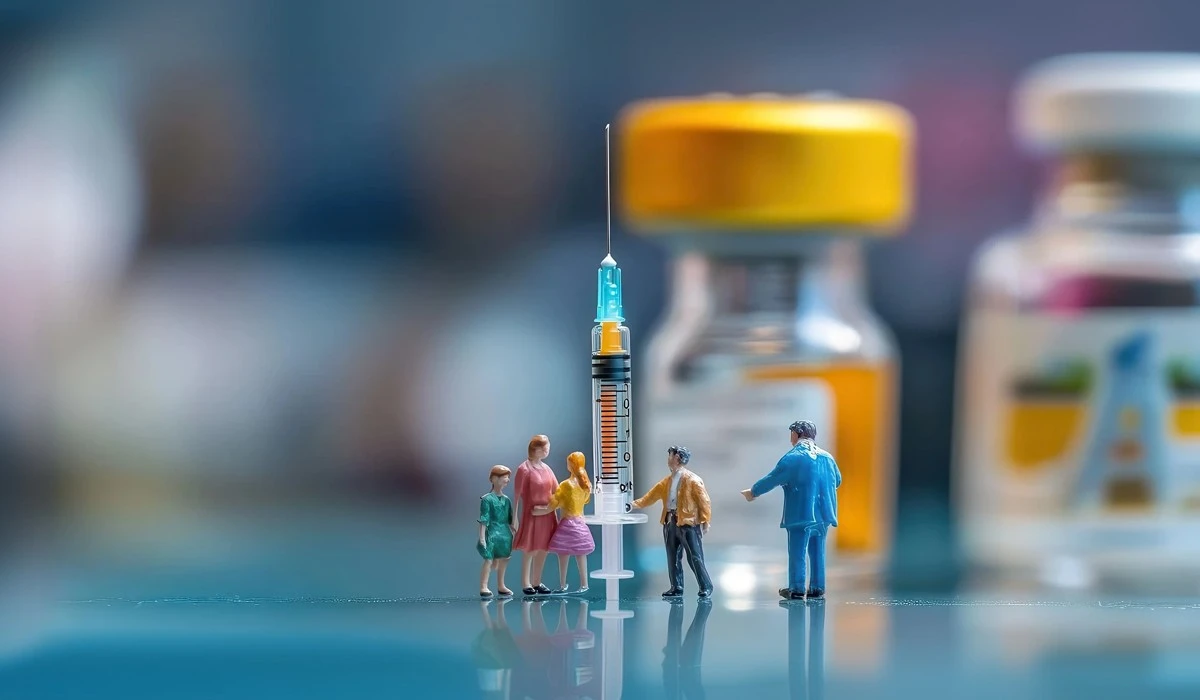
Gene Code Unlocks mRNA Healing
Messenger RNA: The Code That Is Unlocking New Frontiers in Medicine
Scientists are harnessing the power of messenger RNA, a fundamental biological molecule, to create innovative vaccines and therapies. This technology, refined over decades, now stands at the forefront of medical advancement, offering potential solutions for infectious diseases, cancer, and uncommon genetic conditions. Understanding its journey and capabilities reveals a new era in healthcare.
The Science of Cellular Instruction
Messenger RNA, or mRNA, is a vital molecule present in every living cell. Its primary role involves transmitting genetic instructions from DNA, housed within the cell's nucleus, to the cellular machinery responsible for protein synthesis. This process is fundamental to life, as proteins perform a vast array of functions necessary for the body to operate. An mRNA molecule carries the blueprint for a specific protein. After the cell uses these instructions to manufacture the protein, the mRNA molecule naturally degrades. This transient nature is a key characteristic. The information flow is one-way from DNA to mRNA to protein.
Importantly, mRNA from external sources like vaccines does not enter the cell's nucleus; therefore, it cannot alter an individual's DNA. This fact dispels common misconceptions about the technology. Scientists create synthetic mRNA in laboratories to produce vaccines. This laboratory-made mRNA is carefully designed to instruct cells to produce a specific, harmless protein, or even a fragment of a protein, that is characteristic of a pathogen.
A Decades-Long Journey to Breakthrough
The journey of mRNA technology from basic scientific discovery to life-saving medical application spans over half a century. Researchers first identified mRNA in the early 1960s. The initial concept of using mRNA for therapeutic purposes emerged in the 1970s. Further explorations into how it could be delivered into cells took place during that decade. A significant early demonstration occurred in 1989. Scientists successfully delivered designed mRNA, packaged within a fatty envelope known as a liposome, into a cell, resulting in protein expression. A year later, injecting "naked," or unprotected, laboratory-made mRNA into mouse muscle showed that it could instruct living tissue to produce a desired protein. These findings laid the conceptual groundwork for mRNA vaccines.
Despite early enthusiasm, significant technical hurdles slowed progress. A major challenge involved the rapid degradation of mRNA in the body before it could deliver its instructions. Additionally, early forms of synthetic mRNA often triggered unwanted inflammatory responses. The solution to the degradation and delivery problem arrived through advancements in nanotechnology, specifically the development of lipid nanoparticles (LNPs). These tiny fat-based particles encapsulate the mRNA, protecting it and facilitating its entry into cells.
Crucial contributions came from Katalin Karikó and Drew Weissman. Their work, undertaken in the mid-2000s at the University of Pennsylvania, proved pivotal. They discovered that modifying nucleosides, the building blocks of mRNA, could reduce inflammatory reactions and increase protein production from the introduced mRNA. This breakthrough, published in 2005, eliminated critical obstacles to the clinical application of mRNA. The first human clinical trial using mRNA directly injected into the body for cancer cells reported results in 2008. These cumulative efforts set the stage for the technology's rapid deployment when the COVID-19 pandemic emerged.
How mRNA Vaccines Prime the Immune System
mRNA vaccines operate by providing the body's cells with temporary instructions to produce a specific, harmless piece of a pathogen, such as a virus. In the case of COVID-19 vaccines, this is typically a part of the "spike protein" found on the exterior of the SARS-CoV-2 virus. The vaccine, containing mRNA strands encased in lipid nanoparticles, is usually administered into the upper arm muscle. These nanoparticles protect the mRNA and help it enter muscle cells and also some immune cells.
Once inside the cells, the cellular machinery, specifically ribosomes, reads the mRNA's instructions and manufactures the designated protein fragment. The cell then breaks down and removes the mRNA; it does not linger in an individual's system. The newly made protein fragments are then presented externally by these cells. The immune system recognises these protein fragments as foreign, triggering a defensive reaction. This reaction involves activating various immune cells, including B-cells, which generate antibodies, and T-cells, which can identify and destroy infected cells. Antibodies specifically target the foreign protein, marking it for obliteration. Crucially, this process establishes an immunological “recollection.” If the body encounters the actual virus later, the immune system can swiftly recognise the familiar protein and launch a rapid, robust defence, aiding in preventing severe illness.
Image Credit - Freepik
Current Landscape of Approved mRNA Vaccines
The global COVID-19 pandemic served as a catalyst, dramatically accelerating the development and approval of mRNA vaccines. The Pfizer-BioNTech (BNT162b2) and Moderna (mRNA-1273) COVID-19 vaccines were among the first to receive emergency use authorisation and subsequent full approval in many countries. This marked a historic milestone for the technology. These vaccines demonstrated high efficacy in preventing severe disease. The UK's Medicines and Healthcare products Regulatory Agency (MHRA) notably became the first global medicines regulator to approve an mRNA vaccine in December 2020.
Building on this success, mRNA technology has expanded to address other respiratory viruses. An mRNA vaccine for Respiratory Syncytial Virus (RSV), specifically mRNA-1345 by Moderna, has received FDA approval, offering protection for older adults. Pfizer also has mRNA vaccine candidates, including for influenza and shingles, in development. The rapid adaptability of mRNA technology is a significant advantage. It allows for quicker updates to vaccine formulations in response to new viral variants. This contrasts with traditional vaccine manufacturing, which often requires more time-consuming processes like growing viruses in cell cultures or eggs. Regulatory bodies worldwide continue to evaluate new mRNA vaccine candidates for various infectious diseases. This underscores the platform's growing importance in public health. Ongoing research focuses on further refining these vaccines to enhance their efficacy, broaden their applicability, and improve their storage and distribution requirements.
Navigating Concerns and Ensuring Safety
The rapid introduction and widespread use of mRNA vaccines, particularly during the COVID-19 pandemic, understandably led to public questions and concerns regarding their safety. One common misconception is that mRNA vaccines can alter a person's DNA. Health authorities like the US Centers for Disease Control and Prevention (CDC) have consistently clarified that mRNA from vaccines does not enter the cell's nucleus, where DNA is stored. Therefore, it cannot interact with or change an individual's genetic material. The mRNA molecules themselves are also short-lived. They degrade naturally after a brief period following instruction delivery.
Like all vaccines, mRNA vaccines can cause side effects, such as muscle aches, fatigue, or flu-like symptoms. These are generally mild to moderate. They indicate that the immune system is responding to the vaccine as intended. Serious side effects are rare. Rare potential side effects have included myocarditis. This condition involves an inflammation of the heart muscle. Pericarditis, which is an inflammation of the sac surrounding the heart, has also been reported, particularly in younger males, following mRNA COVID-19 vaccination. However, regulatory agencies and health experts have determined that the benefits of vaccination in preventing severe COVID-19, long COVID, and associated complications far outweigh these rare risks. Extensive safety monitoring systems worldwide continue to track vaccine safety. Mortality data reviews have not detected unusual patterns suggesting long-term safety concerns from mRNA COVID-19 vaccines. The technology has undergone rigorous testing phases.
Image Credit - Freepik
Addressing Vaccine Scepticism and Misinformation
Despite the scientific consensus on the safety and efficacy of approved mRNA vaccines, scepticism and misinformation remain significant challenges. Concerns regarding the speed of development during the pandemic, coupled with the novelty of the widespread use of mRNA technology, contributed to public distrust in some quarters. Social media platforms have unfortunately played a role in amplifying inaccurate information and conspiracy theories about these vaccines.
Public health organisations and scientific bodies continue to actively combat misinformation. They provide clear, accessible, and evidence-based information. Addressing public concerns transparently is crucial for maintaining trust in vaccination programmes. Research indicates that negative sentiment towards mRNA vaccines has been prevalent in online discussions. These discussions are often fuelled by alleged severe side effects, rumours, and outright falsehoods. The political landscape has also, at times, complicated public perception. Some figures question the technology without robust scientific backing. Overcoming vaccine hesitancy requires ongoing educational efforts, community engagement, and a commitment from credible sources to debunk myths and provide factual context. Understanding the rigorous approval processes and continuous safety monitoring is vital for informed decision-making.
The Promise of mRNA in Influenza Prevention
Influenza, commonly known as the flu, presents a recurring global health challenge. Seasonal epidemics cause significant illness and mortality. Traditional flu vaccine production is a lengthy process, typically taking at least six months. Scientists must select which influenza virus strains the vaccine will target many months before the flu season begins. This selection is based on surveillance of circulating viruses. This predictive approach can sometimes lead to a mismatch between the vaccine strains and the dominant strains actually causing illness, reducing vaccine effectiveness.
mRNA technology offers a potentially transformative solution to these challenges. The capacity for rapid design and quicker manufacturing of mRNA vaccines, compared to traditional flu shots – potentially in as little as 60 to 120 days – means scientists might delay their decision longer to identify the most relevant circulating flu strains. This closer alignment could significantly improve vaccine effectiveness each season. Several companies, including Pfizer and Moderna, are actively developing mRNA-based influenza vaccines. Some candidates are in late-stage clinical trials. Researchers also hope that mRNA technology might lead to more robust immune responses. Potentially, it could even lead to combination vaccines that could protect against multiple respiratory viruses, such as flu and COVID-19, in a single shot. The adaptability and speed of mRNA platforms are key to this ongoing innovation.
Pioneering mRNA Vaccines for Other Infectious Diseases
The success of mRNA vaccines against COVID-19 and the advancements in RSV and influenza candidates have spurred intensive research into their application for a wide range of other infectious diseases. Clinical trials are currently underway for mRNA vaccines targeting viruses such as HIV, Zika virus, cytomegalovirus (CMV), Epstein-Barr virus (EBV), herpes simplex virus (HSV), chikungunya, Nipah virus, rabies, and varicella-zoster virus (which causes chickenpox and shingles). Each of these pathogens presents unique challenges that traditional vaccine approaches have struggled to overcome.
For instance, the HIV virus is notorious for its rapid mutation rate and its ability to shield critical parts from the immune system. This makes vaccine development exceedingly difficult. mRNA technology's capacity for rapid design iteration and its ability to elicit broad and potent immune responses offer new hope. Similarly, researchers are exploring mRNA vaccines for diseases like malaria, which requires a robust immune response to be effective. While some earlier mRNA vaccine candidates, such as one for Zika (mRNA-1325), showed poor immunogenicity in trials, and another for RSV (mRNA-1365) faced a clinical hold due to safety concerns, these instances provide valuable learning for optimising future designs. The field is continuously evolving. Ongoing efforts aim to improve antigen selection, delivery mechanisms, and overall vaccine performance across diverse infectious agents.
Image Credit - Freepik
A New Dawn for Cancer Treatment: mRNA Immunotherapies
Beyond infectious diseases, cancer immunotherapy represents one of the most exciting frontiers for mRNA technology. The core idea is to use mRNA to instruct a patient's cells to produce proteins that are specific to their cancer cells. These are known as tumour-associated antigens or neoantigens. Once these antigens are produced and presented to the body's defence mechanisms, a targeted immune reaction can be initiated. This process trains the system to recognise and attack the cancer cells. This approach offers the potential for highly personalised cancer treatments.
Numerous clinical trials are investigating mRNA cancer vaccines for various malignancies. These include melanoma, lung cancer, pancreatic cancer, prostate cancer, breast cancer, ovarian cancer, colon cancer, and glioblastoma. Some approaches involve individualised vaccines. The mRNA is designed based on the unique genetic makeup of a patient's specific tumour. For example, BioNTech is developing individualised neoantigen-specific immunotherapies. These have shown promise in early trials for melanoma when combined with other treatments like immune checkpoint inhibitors. Moderna, in partnership with Merck, is also advancing personalised cancer vaccine candidates. Researchers are also exploring using mRNA to encode immune-stimulating agents like cytokines. These can enhance the body's anti-tumour response when used alongside other cancer therapies. Although still at a nascent phase of development, the progress is significant.
Tackling Rare Genetic Disorders with mRNA
mRNA technology holds considerable promise for treating rare genetic disorders. Many of these arise from mutations that prevent the body from producing a crucial functional protein. Unlike gene editing techniques such as CRISPR-Cas9, which involve permanent alterations to DNA, mRNA therapies offer a transient approach. They deliver the genetic instructions for the missing or faulty protein. This allows the patient's cells to temporarily produce the correct version. This can be particularly advantageous for conditions where a reversible or regulatable therapy is preferred.
Cystic fibrosis serves as a compelling example. This condition results from mutations in the CFTR gene. This leads to a defective protein and the production of viscous, adherent mucus which damages the lungs and other organs. Researchers are actively developing mRNA therapies designed to deliver correct instructions for the CFTR protein. They aim to restore normal cell function. Moderna has partnered with Vertex for such therapies. Similarly, investigations are underway for rare metabolic disorders like methylmalonic acidemia and propionic acidemia. In these conditions, enzyme deficiencies disrupt normal metabolic processes. mRNA therapies could potentially encode functional versions of these missing enzymes, restoring metabolic balance. The ability to precisely instruct cells to produce specific proteins opens new therapeutic avenues for many challenging genetic conditions.
Pancreatic Cancer and Cystic Fibrosis: Case Studies in mRNA Potential
Recent research highlights the specific therapeutic potential of mRNA technology in challenging diseases like pancreatic cancer and cystic fibrosis. An early-phase (Phase I) clinical trial for a trial mRNA preventative for pancreatic cancer produced encouraging outcomes. The study, published in Nature, showed that the preventative, administered after surgery, stimulated a defence reaction in certain participants. Those participants demonstrating this defence reaction had extended periods free from their cancer returning compared to participants lacking such a reaction. While these findings are from a small study (16 patients) and require further validation in larger trials, they represent a hopeful step forward for a notoriously difficult-to-treat cancer.
In the realm of cystic fibrosis, a genetic disorder leading to excessively thick mucus, mRNA aims to supply the code for a functional version of the faulty CFTR protein. The goal is to restore the protein's function, thereby returning mucus to a healthier state. Another recent publication demonstrated that, in primate subjects, an inhaled form of mRNA treatment could stimulate the production of a protein crucial for the development of cilia. Cilia are the minute, hair-like projections that line respiratory passages and facilitate the clearance of mucus. These cilia malfunction in a debilitating respiratory ailment identified as primary ciliary dyskinesia. Such targeted mRNA delivery systems are crucial for treating lung-specific diseases. These examples illustrate the diverse strategies researchers are employing to harness mRNA for therapeutic benefit.
Image Credit - Freepik
Innovations in mRNA Delivery: Beyond Lipid Nanoparticles
The success of current mRNA vaccines largely relies on lipid nanoparticles (LNPs) as delivery vehicles. These fatty shells protect the fragile mRNA from degradation and help it enter cells. However, the field is actively exploring alternative and improved delivery systems. The aim is to enhance efficacy, targeting, and stability, and to reduce potential side effects. Researchers are investigating various non-lipid nanoparticle carriers. These include polymer-based systems (polyplexes), peptides, protamine-based systems, dendrimers, and even biologically derived exosomes.
Polymer-based delivery systems, for example, use cationic polymers that interact with the negatively charged mRNA to form protective complexes. These offer versatility in design. However, they can sometimes have lower transfection efficiency or potential toxicity compared to lipids. Other strategies focus on modifying LNPs themselves to target specific cells or tissues more effectively. This could be crucial for therapies aimed at organs beyond the liver, where LNPs tend to accumulate. Innovations like lyophilization (freeze-drying) are being explored to improve vaccine stability at higher temperatures. This could potentially reduce reliance on ultra-cold storage. Furthermore, advancements in creating cell-specific LNPs and designing novel ionizable lipids are pushing the boundaries of mRNA delivery. The goal is more precise and efficient therapeutic action.
The Future of Influenza Vaccines: Enhanced Speed and Precision
The traditional method for producing annual influenza vaccines involves a lengthy process. Global health organisations monitor circulating flu strains throughout the year. Based on these observations, a recommendation for the vaccine composition for the upcoming flu season is typically made in February for the Northern Hemisphere. However, by the time the vaccine is manufactured and distributed months later, the prevalent flu strains may have shifted. This can potentially reduce the vaccine's effectiveness. This is often referred to as vaccine mismatch.
mRNA technology offers a significant advantage here due to its rapid development and manufacturing capabilities. Instead of relying on growing viruses, mRNA vaccines use the virus's genetic code. This code can be synthesized and incorporated into vaccines much faster. This speed could allow scientists to delay the decision on vaccine composition until closer to the flu season. This enables a better match with the actual circulating strains. Pfizer, for instance, initiated a Phase 3 clinical trial for an mRNA flu vaccine candidate. The aim is faster development and more accurate strain matching. The potential for improved efficacy and quicker response to evolving flu viruses positions mRNA as a game-changer for annual influenza prevention.
Expanding Horizons: mRNA for Autoimmune and Cardiovascular Diseases
The versatility of mRNA technology extends its potential application to complex conditions such as autoimmune disorders and cardiovascular diseases. In autoimmune diseases like Type 1 diabetes, the immune system mistakenly attacks the body's own tissues. mRNA therapies could theoretically be designed to modulate or retrain the immune system. For example, by instructing cells to produce specific proteins that promote tolerance or downregulate harmful immune responses. This represents a sophisticated approach to restoring immune balance, moving beyond simple suppression.
For cardiovascular diseases, researchers are exploring how mRNA could be utilised to promote tissue repair after events like a heart attack. It could also deliver therapeutic proteins that could improve heart function or reduce plaque formation in blood vessels. Regenerative medicine is another area where mRNA could promote healing and tissue repair following injury or disease. While research in these areas is generally at an earlier stage compared to infectious disease vaccines or cancer immunotherapies, the fundamental principle of using mRNA to direct cellular protein production opens up numerous therapeutic possibilities. The ability to transiently express specific proteins could offer targeted interventions with potentially fewer side effects than systemic drugs. These applications highlight the broad therapeutic landscape mRNA technology may address in the coming years.
Image Credit - Freepik
Personalised Medicine: Tailoring Treatments with mRNA
A significant aspiration for mRNA technology lies in its potential to revolutionise personalised medicine. Because mRNA can be designed to encode virtually any protein, treatments could be tailored to an individual patient's specific genetic makeup, immune profile, or disease characteristics. This is particularly relevant in oncology. Cancer cells can vary greatly from person to person and even within the same tumour. Personalised cancer vaccines, designed based on the unique neoantigens present on a patient's tumour cells, are a prime example of this approach.
Beyond cancer, mRNA could enable customised therapies for rare genetic disorders. It could provide the specific missing or defective protein instructions unique to that individual. The rapid design and iteration capabilities of mRNA make it well-suited for creating bespoke treatments. Some envision a future where health assessments could lead to real-time, mRNA-based therapies tailored to an individual's evolving biological needs. While challenges in scaling up individualised manufacturing and ensuring cost-effectiveness exist, the prospect of moving away from "one-size-fits-all" medicine towards highly specific, patient-centric interventions is a driving force behind much mRNA research. This paradigm shift could transform how many complex diseases are managed.
Manufacturing and Scalability: Meeting Global Demand
The COVID-19 pandemic highlighted the remarkable ability to rapidly scale up mRNA vaccine production to meet unprecedented global demand. mRNA vaccine manufacturing is generally considered less complex and faster than traditional vaccine production methods. These often involve cell cultures or egg-based processes. The cell-free production of RNA can be readily scaled for mass manufacturing. This efficiency is a key advantage, particularly in responding to emerging infectious disease threats.
However, the evolution of mRNA technology towards personalised therapies for conditions like cancer or rare diseases introduces new manufacturing challenges. Instead of producing millions of doses of a single vaccine, manufacturers may need to produce many small, individualised batches. This shift requires adapting manufacturing processes to offer a spectrum of production scales. This ranges from large-scale for pandemic preparedness to numerous small-scale bioreactors for personalised treatments. Ensuring quality control and regulatory consistency across decentralised or smaller manufacturing sites will be crucial. Continued investment in infrastructure and process optimisation is necessary to support the diverse and expanding applications of mRNA therapies. Innovations in areas like lyophilization for improved stability could also simplify global distribution and reduce reliance on complex cold chains.
The Regulatory Landscape and Future Outlook
The successful rapid development and deployment of mRNA vaccines during the COVID-19 pandemic were facilitated by emergency use authorisations and accelerated regulatory pathways. As mRNA technology matures and expands into new therapeutic areas like cancer and rare diseases, navigating the regulatory landscape remains a key consideration. Regulatory bodies worldwide, including the MHRA in the UK, the FDA in the US, and the European Medicines Agency (EMA), are continually adapting their frameworks to evaluate these novel therapies.
Establishing clear guidelines for the development, manufacturing, and quality control of diverse mRNA products is essential. This applies from widespread vaccines to highly personalised medicines. For personalised cancer vaccines or treatments for rare diseases, clinical trial designs and approval processes may need specific considerations. Challenges include ensuring the long-term stability of mRNA products. Addressing the costs associated with advanced delivery systems like LNPs, which can impact global access, is also a challenge. Despite these hurdles, the outlook for mRNA technology is overwhelmingly positive. Continued collaboration between academia, industry, and regulatory agencies will be vital to fully realise the transformative potential of mRNA in treating a vast array of human diseases and improving public health globally. The ongoing research and development promise a future where mRNA-based medicines play an increasingly significant role.
Recently Added
Categories
- Arts And Humanities
- Blog
- Business And Management
- Criminology
- Education
- Environment And Conservation
- Farming And Animal Care
- Geopolitics
- Lifestyle And Beauty
- Medicine And Science
- Mental Health
- Nutrition And Diet
- Religion And Spirituality
- Social Care And Health
- Sport And Fitness
- Technology
- Uncategorized
- Videos